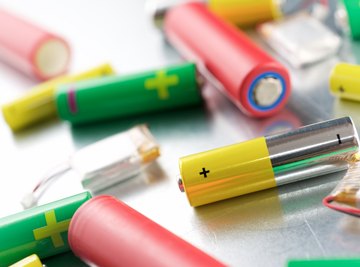
You've probably encountered batteries going flat, which is a nuisance if you're trying to use them in electronics devices. The cell chemistry of batteries can tell you properties of how they work including how they go flat.
Cell Chemistry of Batteries
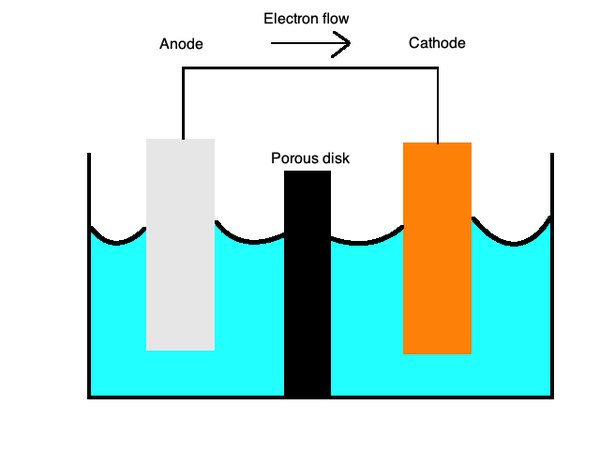
When the electrochemical reaction of a battery depletes the materials, the battery goes flat. This generally happens after a long time of battery use.
Batteries generally use primary cells, a type of galvanic cell that uses two different metals in a liquid electrolyte to allow the transfer of charge between them. Positive charges flow from the cathode, built with cations or positively charged ions such as copper, to the anode, with anions or negatively charged ions such as zinc.
Tips
Batteries go flat as a result of the electrolyte's chemicals drying up within the battery. In the case of alkaline batteries, this is when all of the manganese dioxide has been converted. At this stage the battery is flat.
To remember this relationship, you can remember the word "OILRIG." This tells you that oxidation is loss (“OIL”) and reduction is gain (“RIG”) of electrons. The mnemonic for anodes and cathodes is "ANOX REDCAT" to remember that the "ANode" is used with "OXidation" and "REDuction" occurs at the "CAThode."
Primary cells can also work with individual half-cells of different metals in an ionic solution connected by a salt bridge or a porous membrane. These cells provide batteries with a myriad of uses.
Alkaline batteries, which specifically use the reaction between a zinc anode and a magnesium cathode, are used for flashlights, portable electronic devices and remote controls. Other examples of popular battery elements include lithium, mercury, silicon, silver oxide, chromic acid and carbon.
Engineering designs can take advantage of the way batteries go flat to conserve and re-use energy. Low-cost household batteries generally use carbon-zinc cells designed such that, if the zinc undergoes galvanic corrosion, a process in which a metal corrodes preferentially, the battery may produce electricity as part of a closed electron circuit.
At what temperature do batteries explode? The cell chemistry of lithium-ion batteries means these batteries start chemical reactions that result in their explosion at around 1,000 °C. The copper material inside of them melts which causes the internal cores to break.
History of the Chemical Cell
In 1836 British chemist John Frederic Daniell constructed the Daniell cell in which he used two electrolytes, instead of just one, to let hydrogen produced by one to be consumed by the other. He used zinc sulfate instead of sulfuric acid, common practice of batteries of the time.
Before then, scientists used voltaic cells, a type of chemical cell that uses a spontaneous reaction, which lost power at fast rates. Daniell used a barrier between the copper and zinc plates to prevent excess hydrogen from bubbling and stop the battery from wearing down quickly. His work would lead to innovations in telegraphy and electrometallurgy, the method of using electrical energy to produce metals.
How Rechargeable Batteries Go Flat
Secondary cells, on the other hand, are rechargeable. The rechargeable battery, also called storage battery, secondary cell or accumulator, stores charge over time as the cathode and anode are connected in a circuit with one another.
When charging, the positive active metal such as nickel oxide hydroxide becomes oxidized, creating electrons and losing them, while the negative material such as cadmium is reduced, capturing electrons and gaining them. The battery uses charging-discharging cycles using a variety of sources including alternating current electricity as an external voltage source.
Rechargeable batteries can still go flat after repeated use because the materials involved in the reaction lose their ability to charge and re-charge. As these battery systems wear out, there are different ways the batteries go flat.
As batteries are used routinely, some of them such as lead-acid batteries may lose the ability to recharge. The lithium of lithium-ion batteries may become reactive lithium metal which can't re-enter the charge-discharge cycle. Batteries with liquid electrolytes may decrease in their moisture due to evaporation or overcharging.
Applications of Rechargeable Batteries
These batteries are generally used in automobiles starters, wheelchairs, electric bicycles, power tools and battery storage power stations. Scientists and engineers have studied their use in hybrid internal combustion-battery and electric vehicles to become more effective in their power use and last longer.
The rechargeable lead-acid battery breaks water molecules (H2O) into aqueous hydrogen solution (H+) and oxide ions (O2-) which produces electrical energy from the broken bond as the water loses its charge. When the aqueous hydrogen solution reacts with these oxide ions, the strong O-H bonds are used to power the battery.
Physics of Battery Reactions
This chemical energy powers a redox reaction that converts high-energy reactants to lower-energy products. The difference between the reactants and products lets the reaction happen and forms an electrical circuit when the battery is hooked up by converting chemical energy into electrical energy.
In a galvanic cell, the reactants, such as metallic zinc, have a high free energy that lets the reaction occur spontaneously without external force.
The metals used in the anode and cathode have lattice cohesive energies that can drive the chemical reaction. The lattice cohesive energy is the energy required to separate the atoms that make the metal from one another. Metallic zinc, cadmium, lithium and sodium are often used because they have high ionization energies, the minimum energy required to remove electrons from an element.
Galvanic cells driven by ions of the same metal can use differences in free energy to cause Gibbs free energy to drive the reaction. The Gibbs free energy is another form of energy used to calculate the amount of work a thermodynamic process uses.
In this case, the change in standard Gibbs free energy Go drives the voltage, or electromotive force Eo in volts, according to the equation
in which ve is the number of electrons transferred during the reaction and F is Faraday's constant (F = 96485.33 C mol−1).
The ΔrGo indicates the equation uses the change in Gibbs free energy (ΔrGo = Gfinal - Ginitial). Entropy increases as the reaction uses the available free energy. In the Daniell cell, the lattice cohesive energy difference between zinc and copper account for most of the Gibbs free energy difference as the reaction occurs. ΔrGo = -213 kJ/mol, which is the difference in Gibbs free energy of the products and that of the reactants.
Voltage of a Galvanic Cell
If you separate the electrochemical reaction of a galvanic cell into the half reactions of oxidation and reduction processes, you can sum the corresponding electromotive forces to obtain the total voltage difference used in the cell.
For example, a typical galvanic cell may use CuSO4 and ZnSO4 with standard potential half reactions as: Cu2+ + 2 e− ⇌ Cu with a corresponding electromotive potential Eo = +0.34 V and Zn2+ + 2 e− ⇌ Zn with potential Eo = −0.76 V.
For the overall reaction, Cu2+ + Zn ⇌ Cu + Zn2+ , you may "flip" the half reaction equation for zinc while flipping the sign of the electromotive force to obtain Zn ⇌ Zn2+ + 2 e− with Eo = 0.76 V. The overall reaction potential, the sum of the electromotive forces, is then +0.34 V − (−0.76 V) = 1.10 V.
References
About the Author
S. Hussain Ather is a Master's student in Science Communications the University of California, Santa Cruz. After studying physics and philosophy as an undergraduate at Indiana University-Bloomington, he worked as a scientist at the National Institutes of Health for two years. He primarily performs research in and write about neuroscience and philosophy, however, his interests span ethics, policy, and other areas relevant to science.