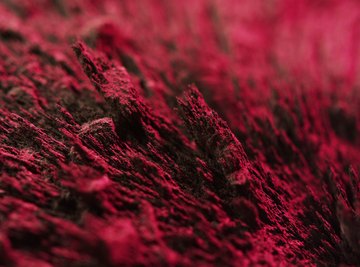
Almost everyone is familiar with a basic magnet and what it does, or can do. A small child, if a given a few moments of play and the right mix of materials, would quickly recognize that certain kinds of things (which the child will later identify as metals) are pulled toward the magnet while others are unaffected by it. And if the child is given more than one magnet to play with, the experiments will quickly become even more interesting.
Magnetism is a word encompassing a number of known interactions in the physical world that are not visible to the unaided human eye. The two basic types of magnets are ferromagnets, which create permanent magnetic fields around themselves, and electromagnets, which are materials in which magnetism can be temporarily induced when they are placed in an electric field, such as that generated by a coil of current-carrying wire.
If someone asks you the Jeopardy-style question "A magnet is made up of which material?" then you can be confident that there is no single answer – and armed with the information at hand, you'll even be able to explain to your questioner all of the helpful details, including how a magnet is formed.
History of Magnetism
As with so much in physics – for example, gravity, sound and light – magnetism has always "been there," but humankind's ability to describe it and make predictions about it based on experiments and the resulting models and frameworks has progressed throughout the centuries. An entire branch of physics has sprung up around the related concepts of electricity and magnetism, usually called electromagnetics.
Ancient cultures were aware that the lodestone, a rare type of the iron-and-oxygen-containing mineral magnetite (chemical formula: Fe3O4), could attract pieces of metal. By the 11th century, the Chinese had learned that such a stone that happened to be long and thin would orient itself along a north-south axis if suspended in air, paving the way for the compass.
European voyagers making use of the compass noticed that the direction indicating north varied slightly throughout trans-Atlantic journeys. This led to the realization that the Earth itself is essentially a massive magnet, with "magnetic north" and "true north" being slightly different, and different by varying amounts across the globe. (The same applies to true and magnetic south.)
Magnets and Magnetic Fields
A limited number of materials, including iron, cobalt, nickel and gadolinium, manifest strong magnetic effects on their own. All magnetic fields result from electrical charges moving relative to one another. The induction of magnetism in an electromagnet by placing it near a coil of current-carrying wire has been mentioned, but even ferromagnets possess magnetism only because of tiny currents generated at the atomic level.
If a permanent magnet is brought near a ferromagnetic material, the components of individual atoms of iron, cobalt or whatever the material is align themselves with the imaginary lines of influence of the magnet fanning out from its north and south poles, called the magnetic field. If the substance is heated and cooled, the magnetization can be made permanent, though it can also occur spontaneously; this magnetization can be reversed by extreme heat or physical disruption.
No magnetic monopole exists; that is, there is no such thing as a "point magnet," as occurs with point electric charges. Instead, magnets have magnetic dipoles, and their magnetic field lines originate at the north magnetic pole and fan outward before returning to the south pole. Remember, these "lines" are just tools used to describe the behavior of atoms and particles!
Magnetism at the Atomic Level
As emphasized previously, magnetic fields are produced by currents. In permanent magnets, tiny currents are produced by the two types of motion of the electrons in these magnets atoms: Their orbit about the central proton of the atom, and their rotation, or spin.
In most materials, the small magnetic moments created by the motion of the individual electrons of a given atom cancel each other out. When they do not, the atom itself acts like a tiny magnet. In ferromagnetic materials, the magnetic moments not only don't cancel out, but they also align themselves in the same direction, and shift so as to be aligned in the same direction as the lines of an applied external magnetic field.
Some materials have atoms that behave in such a way as to allow them to be magnetized to varying degrees by an applied magnetic field. (Remember, you don't always need a magnet for a magnetic field to be present; a sizable enough electric current will do the trick.) As you'll see, some of these materials want no lasting part of magnetism, whereas others behave in a more wistful way.
Classes of Magnetic Materials
A magnetic-materials list that gives only the names of metals exhibiting magnetism would not be nearly as useful as a list of magnetic materials ordered by the behavior of their magnetic fields and how things operate at the microscopic level. Such a classification system exists, and it separates magnetic behavior into five types.
- Diamagnetism: Most materials exhibit this property, in which the magnetic moments of atoms placed in an external magnetic field align themselves in a direction opposite that of the applied field. Accordingly, the resulting magnetic field opposes the applied field. This "reactive" field, however, is very weak. Because materials with this property are not magnetic in any meaningful sense, the strength of the magnetism is not dependent on temperature.
- Paramagnetism: Materials with this property, such as aluminum, have individual atoms with positive net dipole moments. The dipole moments of neighboring atoms, however, usually cancel each other out, leaving the material as a whole unmagnetized. When a magnetic field is applied, rather than opposing the field outright, the magnetic dipoles of the atoms align themselves incompletely with the applied field, resulting in a weakly magnetized material.
- Ferromagnetism: Materials such as iron, nickel and magnetite (lodestone) have this potent property. As touched upon already, the dipole moments of neighboring atoms align themselves even in the absence of a magnetic field. Their interactions can result in a magnetic field of magnitudes reaching 1,000 tesla, or T (the SI unit of magnetic field strength; not a force but something like one). By comparison, the magnetic field of the Earth itself is 100 million times weaker!
- Ferrimagnetism: Note the difference of a single vowel from the previous class of materials. These materials are usually oxides, and their unique magnetic interactions stem from the fact that the atoms in these oxides are arranged in a crystal "lattice" structure. The behavior of ferrimagnetic materials is very much like that of ferromagnetic materials, but the ordering of the magnetic elements in space is different, leading to different levels of temperature sensitivity and other distinctions.
- Antiferromagnetism: This class of materials is characterized by a peculiar temperature sensitivity. Above a given temperature, called the Neel temperature or TN, the material behaves much like a paramagnetic material. One example of such a material is hematite. These materials are also crystals, but as their name implies, the lattices are organized in such as way that the magnetic dipole interactions are completely canceling when no external magnetic field is present.
References
About the Author
Kevin Beck holds a bachelor's degree in physics with minors in math and chemistry from the University of Vermont. Formerly with ScienceBlogs.com and the editor of "Run Strong," he has written for Runner's World, Men's Fitness, Competitor, and a variety of other publications. More about Kevin and links to his professional work can be found at www.kemibe.com.